From a Cell to a Lung: An Arduous Journey
- Sidharth Raghavan
- Oct 14, 2022
- 7 min read
Updated: Oct 14, 2022
Entangled Strands Podcast Episode 1: Dr. Hans Willem-Snoeck | Building Lungs and the Future of Stem Cell Therapies
A cell enigma, building artificial lungs, and the future of stem cell therapies.
I. A Cell to a Human
Cells are the fundamental building blocks of every living thing on earth. At the dawn of life, there existed only single-celled organisms. They’ve since transformed into intricate human beings that can learn, think, and create. Humans are composed of over 200 different cell types - such as bone, muscle, blood, and nerve - which perform a variety of crucial actions. Thus, it’s clear that the cell’s structure has drastically evolved since it was first forged 3.8 billion years ago. For centuries, the mystery of how a single cell gives rise to a complex human being has baffled scientists. Today we understand much more about this process. In the 1880s, Walter Sutton and Theodor Boveri discovered that the chromosome contains the instructions for the cell. Later, Oswald Avery, Colin MacLeod, and Maclyn McCarty demonstrated that DNA (which is contained in chromosomes), rather than proteins, is responsible for the properties of a cell. Now, many understood where cells received the directions to transform into new cells, but an important part of the story remained missing: all cells contain DNA, so what distinguishes cells that can become new cells from those that cannot, like skin cells?
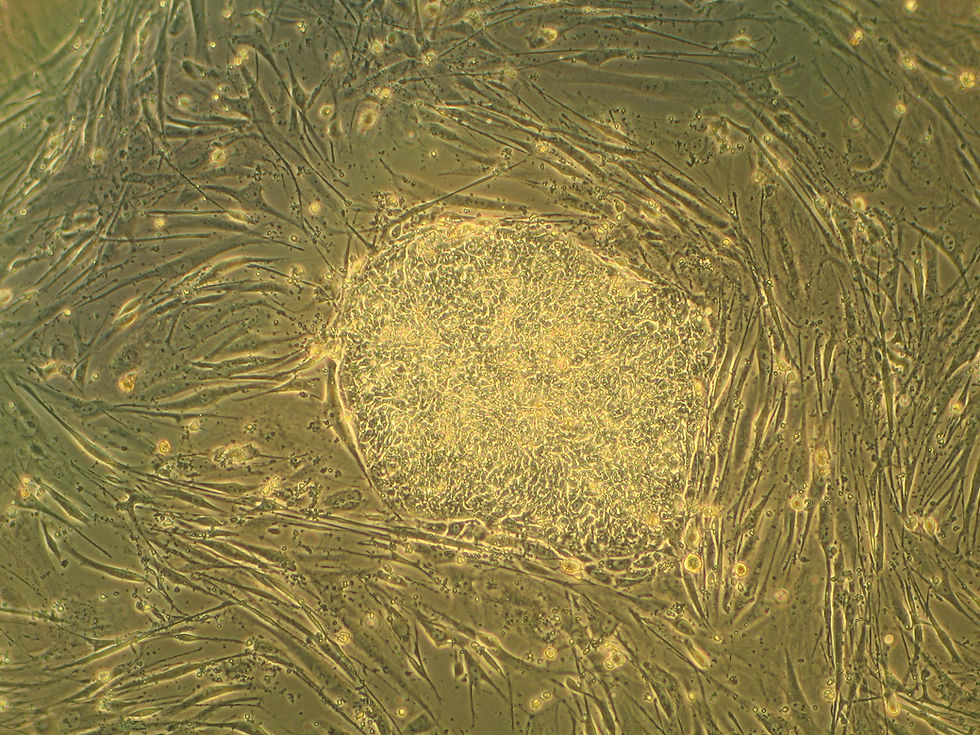
Stem cells were the key to this puzzle. In 1961, Ernest McCulloch and James Till found a peculiar blood cell that could replicate and transform into the different cells that form blood. These cells were later known as hematopoietic (blood) stem cells because they can (1) replicate and (2) differentiate into many blood cell types. But all human cells begin as stem cells at inception in the human embryo. During reproduction, egg and sperm cells are fertilized to form a zygote. The zygote develops into a blastocyst and then an embryo, which contains embryonic stem (ES) cells, prior to implantation in the uterus. These stem cells will differentiate into a myriad of new cells that make up our different tissues and organs.
II. From One, Many
All cells in the human body begin as embryonic stem (ES) cells which are pluripotent, i.e., they can self-renew and differentiate into other, more specific cells. But what causes one cell to transform into a completely different cell? And how does an ES cell become a heart cell that beats to keep us alive? The answer lies in the cell's genome. When differentiation occurs, certain genes inside the cell are activated or deactivated. Any genetic change is based on signals that the cell receives from surrounding tissues and cells. Scientists know how to signal ES cells to differentiate into some cells like heart cells, but this process is complicated and there is much that must be solved to produce these cells commercially. Embryonic stem cells can transform into a plethora of other cells, so finding the genes responsible for a particular cell outcome can be incredibly difficult.
Since McCulloch and Till’s discovery of the stem cell in 1961, stem cell biology has come a long way. However, success can bring additional difficulties. Many ethical concerns with using ES cells, which must come from another person’s embryo, began to surface. As a result, there are now many limitations on the use of ES cells which hampers scientific progress in the field.

In 2006, Shinya Yamanaka made a pivotal discovery that would transform the world. He identified four genes - Oct3/4, Sox2, Klf4, and c-Myc - that are responsible for giving stem cells their pluripotent qualities. Using a retrovirus, he programmed these four genes into skin cells, giving them pluripotency, just like ES cells.
Yamanaka had created induced pluripotent stem (iPS) cells, which are effectively a substitute for ES cells, and what’s more, scientists have an unlimited supply at their disposal since they are not extracted from an embryo. Today, iPS cells are being used to help eliminate immune rejection during transplantation, model diseases, and create gene therapies. Embryonic stem cells come from another person; however, iPS cells can be engineered from the patient, eliminating concerns of immune rejection for a transplant. Finally, it’s also vital that scientists are able to test new treatments without risking them on patients. By injecting certain diseases into iPS-derived cell cultures, scientists can gain a better understanding of how the disease works. These induced pluripotent stem cells now help cure millions around the world and Yamanaka won the Nobel Prize in Medicine, in 2012, for the innovation.
Induced pluripotent stem cells also allow scientists to re-create a human environment inside of a test tube. Perhaps the most exciting stem cell development is building organoids (i.e. tissue cultures derived from stem cells) which can mimic the behaviors of an organ. In this article, I’ll focus on the groundbreaking research of one scientist who is working on growing artificial lungs using pluripotent stem cells.
III. Interview - Dr. Hans-Willem Snoeck
This past June, I had the privilege of interviewing Dr. Hans-Willem Snoeck, a Professor of Medicine at Columbia University studying blood stem cells and the immune system. Dr. Snoeck also focuses on human lung development and disease. Our conversation spanned many areas: we discussed lung development, stem cell therapies, and his beginnings in science. You can listen to our full dialogue on my podcast, Entangled Strands.
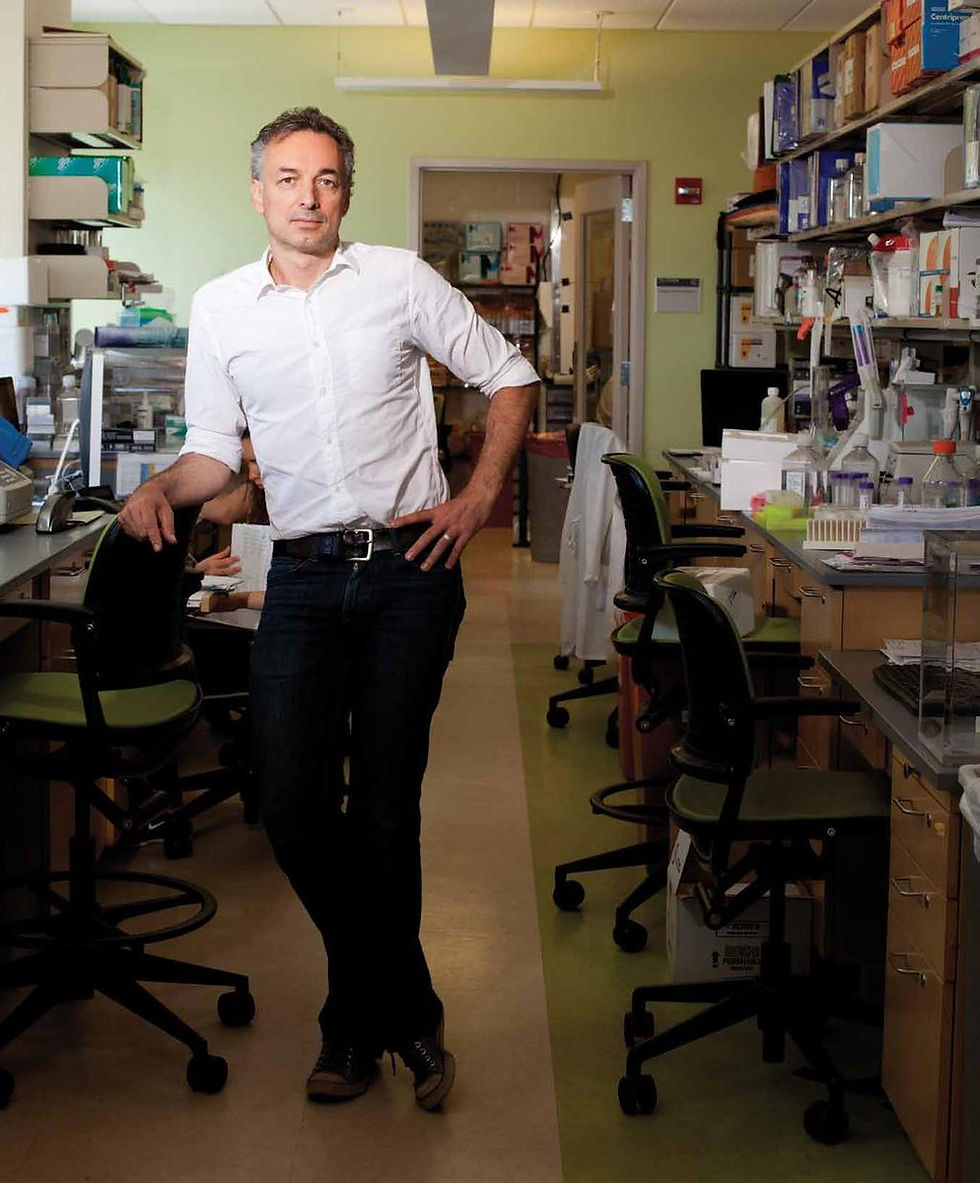
Dr. Snoeck grew up in Belgium at an important scientific juncture: the HIV/AIDs pandemic. He was so fascinated by the virus that he decided that he could best serve humanity by doing science, and more specifically, immunology. Dr. Snoeck soon found himself absorbed by another disease: leukemia. T cells are immune fighter cells that are produced by the thymus (an organ in the upper-chest) during a child’s early years. As one gets older, one’s thymus shrinks and produces less T cells. Unfortunately, chemotherapy and radiation treatments for leukemia destroy a patient’s T cell counts. This would result in a patient being immunocompromised, drastically increasing that patient’s risk of severe infection. Then, Dr. Snoeck had a pivotal idea: could it be possible to grow a transplantable thymus using pluripotent stem cells? After many attempts, the project failed - no thymus cells were differentiated; however, unexpectedly, cells from a familiar organ appeared.
IV. Building Lungs-in-a-Dish
Lung cells found their way into Dr. Snoeck’s thymus culture due to their physical proximity to the thymus, spurring his journey towards creating lungs from pluripotent stem cells. Now, Dr. Snoeck works on building 3D models of the lung, referred to as lung organoids, which can model diseases and may one day become an option for lung transplants. But creating lungs from pluripotent stem cells is no easy feat. How can one identify which genes to modify for the transformation of a cell?
Dr. Snoeck modified human pluripotent stem cells in numerous ways and tested their differentiation in mice with the hope of cultivating lung tissues. Finally, in 2011, he identified chemical factors (which alter certain genes) that are responsible for differentiating an ES or iPS cell into the precursors for lung and airway cells. Two years later, Dr. Snoeck succeeded in the full differentiation of lung and airway cells when he identified more factors responsible for converting the precursor cells into complete cells.
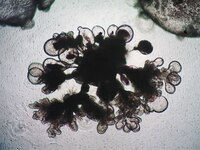
In 2017, Dr. Snoeck successfully performed something that had never been done before: building lung organoids that included branching airways and alveolar structures that closely mimicked the activity of the human lung. What’s more, when the organoid was infected with respiratory syncytial virus (RSV), the lung cells began to swell and shred. Using Dr. Snoeck’s significant innovation, scientists can now test for lung diseases in human lung organoids which closely mimic the real thing. While full artificial lung transplants still remain a fairytale treatment, there are other solutions that may be on the horizon. Lung stem cells could be weaved into a patient’s lung scaffold to help improve their disease condition. The next decade holds immense promise for the future of lung stem cell treatments.
But the beauty of this discovery stretches far beyond an organoid. It’s yet another example of how intricate and elegant our human body is. Dr. Snoeck couldn’t believe his eyes - stem cells can self-organize into a working contraption that can breathe. Self-organization is an ‘emergent’ property of cells - one that single cells do not individually possess - that allows them to communicate with their environment. When cells form groups (tissues), they control the spatial distribution of themselves to help form a specific tissue or organ. However, as we dig deeper into this phenomenon, shades of mystery begin to appear. How does cell communication occur? What causes cells to self-organize? Getting to the bottom of this complex enigma will help unlock the next generation of stem cell engineering by allowing us to exploit cell communication processes.
V. A Bright Future
Decades of scientific research has led to this moment: building living organs in the lab. From McCulloch and Till’s discovery of the stem cell to lung organoids and stem cell therapies, biology has progressed immensely. As science and medicine continue to advance, new frontiers of research are unfurled. Instead of solely focusing on the cell, scientists must take a holistic approach to cell differentiation and communication to understand the numerous sources that can lead to cells taking a certain action.
Induced pluripotent stem cells have been the key to stem cell research over the last ten years and will continue to allow us to push the boundaries of science. Although they are a great option in the lab, it’s worth noting that iPS cells aren’t easily deployable in practice. Differentiating iPS cells is already a hurdle in the lab; thus, manufacturing cells in a Good Manufacturing Practice (GMP) condition will be difficult.
It’s unclear where stem cell innovation will take us next, but there lies a bright and exciting future ahead. Stem cells could one day be used to create artificial organs and tissues: recently, scientists at the Weizmann Institute in Israel successfully engineered a mouse embryo using embryonic stem cells. Each day, we’re getting closer to deciphering the magnificent puzzle that lies inside of us.
Many thanks to Dr. Hans-Willem Snoeck for participating in the interview. You can listen to our full conversation here. Thanks for reading.
Support Entangled Strands on Spotify, Apple Podcasts, and YouTube!
Komentāri